
Quantum Computer Odyssey: Unveiling the Inner Core
Prepare for a mesmerising journey into the frigid depths of a mysterious marvel of modern technology
IBM’s quantum computer has a tiny chip, as small as a quarter. These special machines can tackle tough problems that regular computers can’t. The chip is just one piece of a bigger puzzle.
Unlike the laptops we use every day, the stuff that helps the quantum chip work is complicated, like a set of nesting dolls, with lots of tricky connections in a complex contraption.
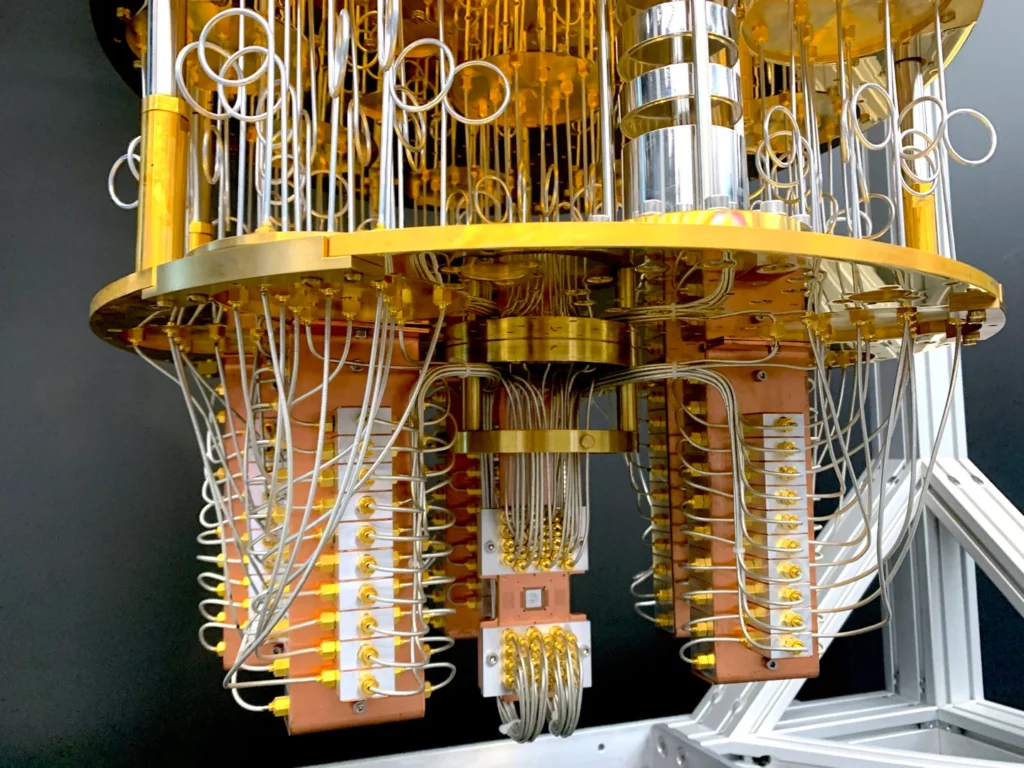
Still, despite its complex structure and bewildering design, a quantum computer is, at its core, a machine that carries out tasks using both its physical parts and its software.
Some of these tasks are quite similar to what regular computers do. If you’re curious to learn how they work, Popular Science had the chance to explore the quantum hub at IBM’s Yorktown Heights, New York campus.
Let’s take a closer look at what’s happening inside, beginning with a concept called the “qubit” (we’ll explain that in a moment) and gradually expanding our view step by step.
That’s cold
To show quantum characteristics, things need to be really tiny or extremely cold. IBM uses a unique structure, resembling an upside-down gold chandelier mixed with steampunk aesthetics, and they call it a “dilution refrigerator.”
This refrigerator keeps their qubits, which are the building blocks of quantum computing, very cold and steady. It’s the system IBM designed for their 50-qubit chip.
Inside this refrigerator, there are several plates, and the closer they are to the bottom, the colder they get. Each plate has a different temperature, with the top one staying at room temperature.
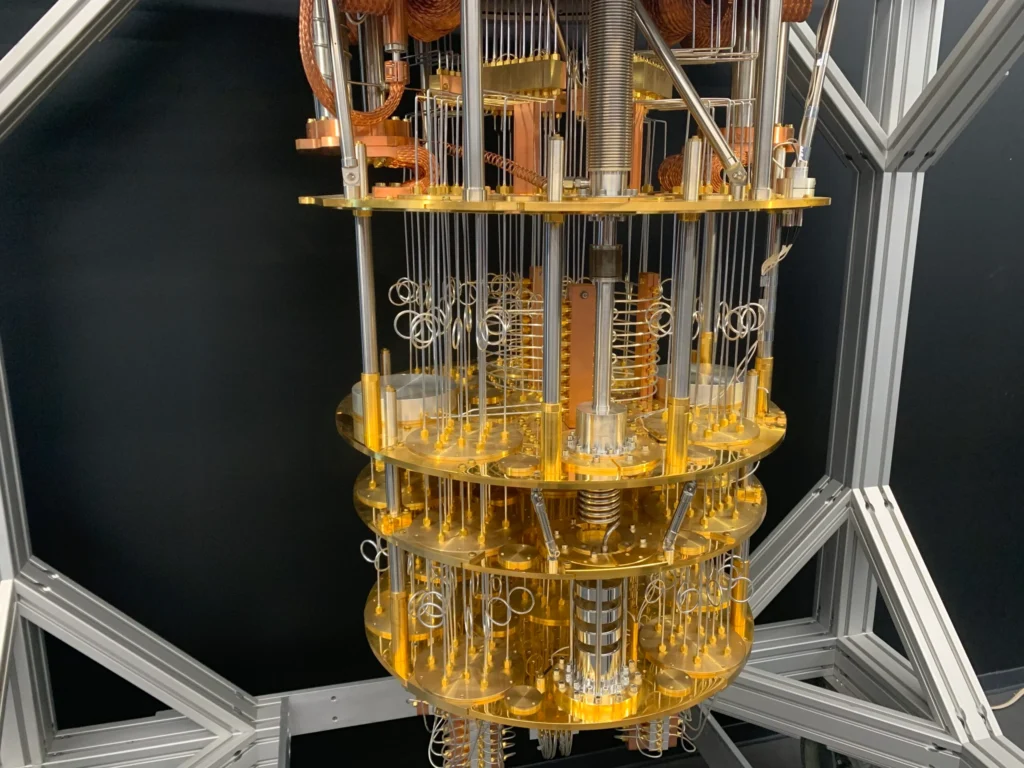
The quantum processor is attached to the coldest plate at the very bottom of the dilution refrigerator, reaching temperatures of about 10 to 15 milli-Kelvin, which is roughly -238 degrees Celsius (-460 degrees Fahrenheit).
The initial cooling stage involves big copper pieces hanging down in the top layer, connected to cold units as part of closed-cycle helium cryocooler.
Additional tubes go into the lower levels, introducing another closed cycle of super cold material, which is a blend of different types of helium isotopes.
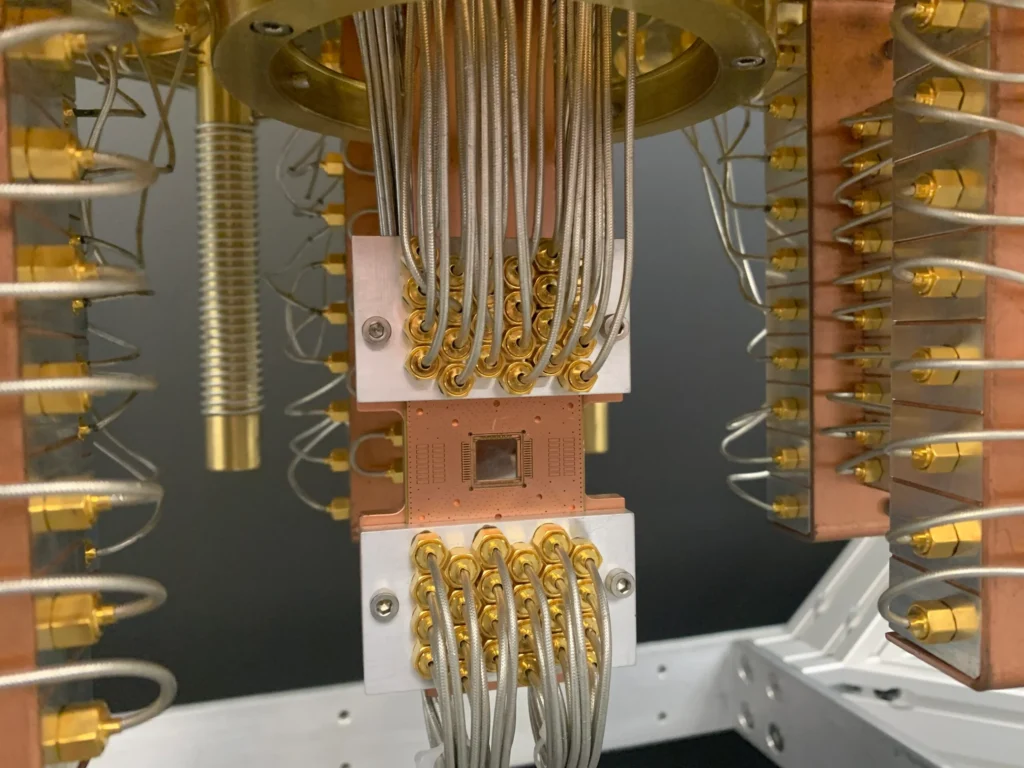
At the rear of the housing structure, you’ll find the hidden support systems for the chandelier-like structure. This includes the gas handling system that helps extremely cold setup, along with pumps & temperature sensors.
Additionally, there are custom-made classical control electronics. When users operate a program using IBM’s quantum cloud service, they’re essentially coordinating a series of gates and their circuits.
These are then transformed into microwave pulses that are properly organised, synchronised, and sent throughout the system to manage the qubits. The readout pulses retrieve the qubits’ states, which are converted back into binary values and given back to the users.
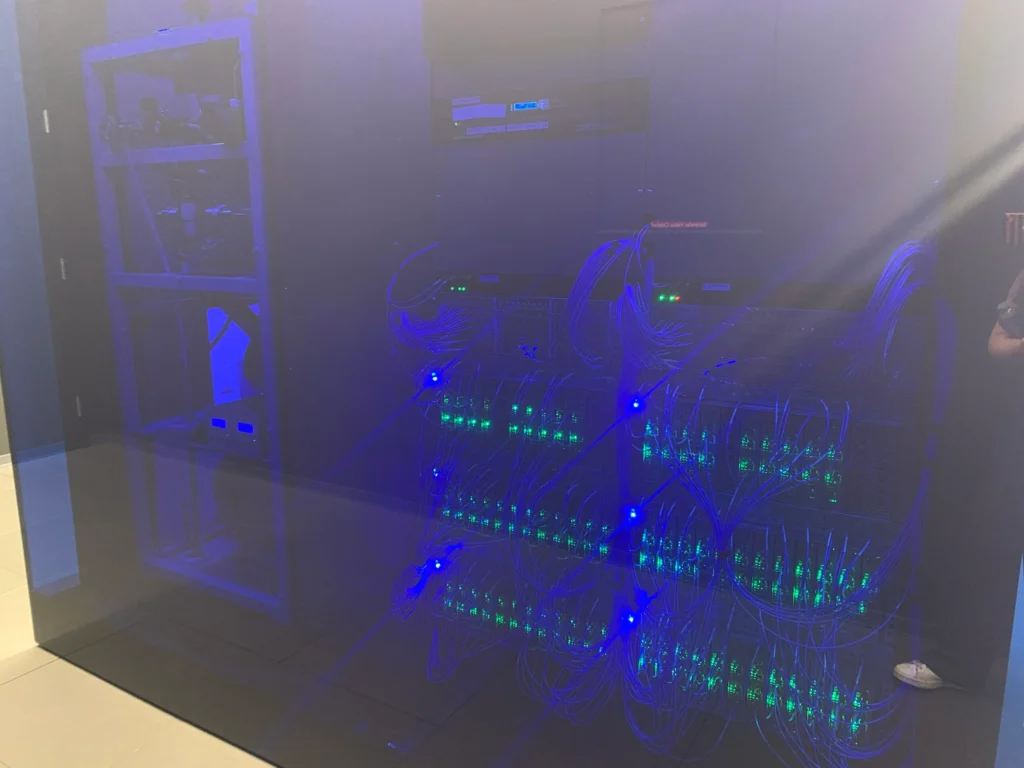
Qubits & an ‘artificial atom’
Regular computers use binary bits, which can be either one or zero to represent information. In the world of quantum computing, information is handled using qubits, which can exist in a combination of both zero and one simultaneously.
This unique property is known as “superposition.” Zaira Nazario, who is the technical lead of theory, algorithms, and applications at IBM Quantum, explains that superposition is a common phenomenon in the real world, like how music is a blend of different frequencies.
Qubits, being waveforms, have an amplitude that can be both zero and one at the same time. This duality includes a phase, and similar to other waves, qubits can interact with each other.
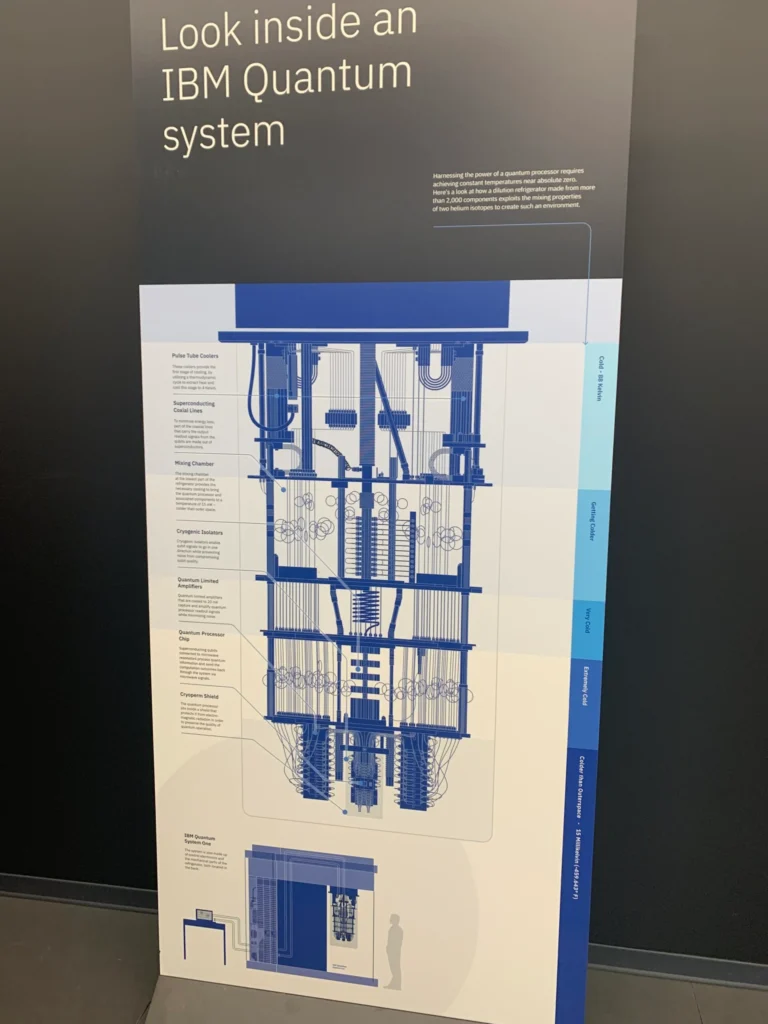
The superconducting qubits are located on the chip and have been assembled into something resembling a printed circuit board. There are wires and coaxial cables attached to the circuit board for handling input and output signals.
In the latest versions of chips with more qubits, IBM has been developing more streamlined designs with integrated components to save space and improve efficiency. A neater setup makes it easier to maintain the right cooling conditions.
At present, it takes approximately 48 hours to fully cool down a quantum computer to the necessary temperatures
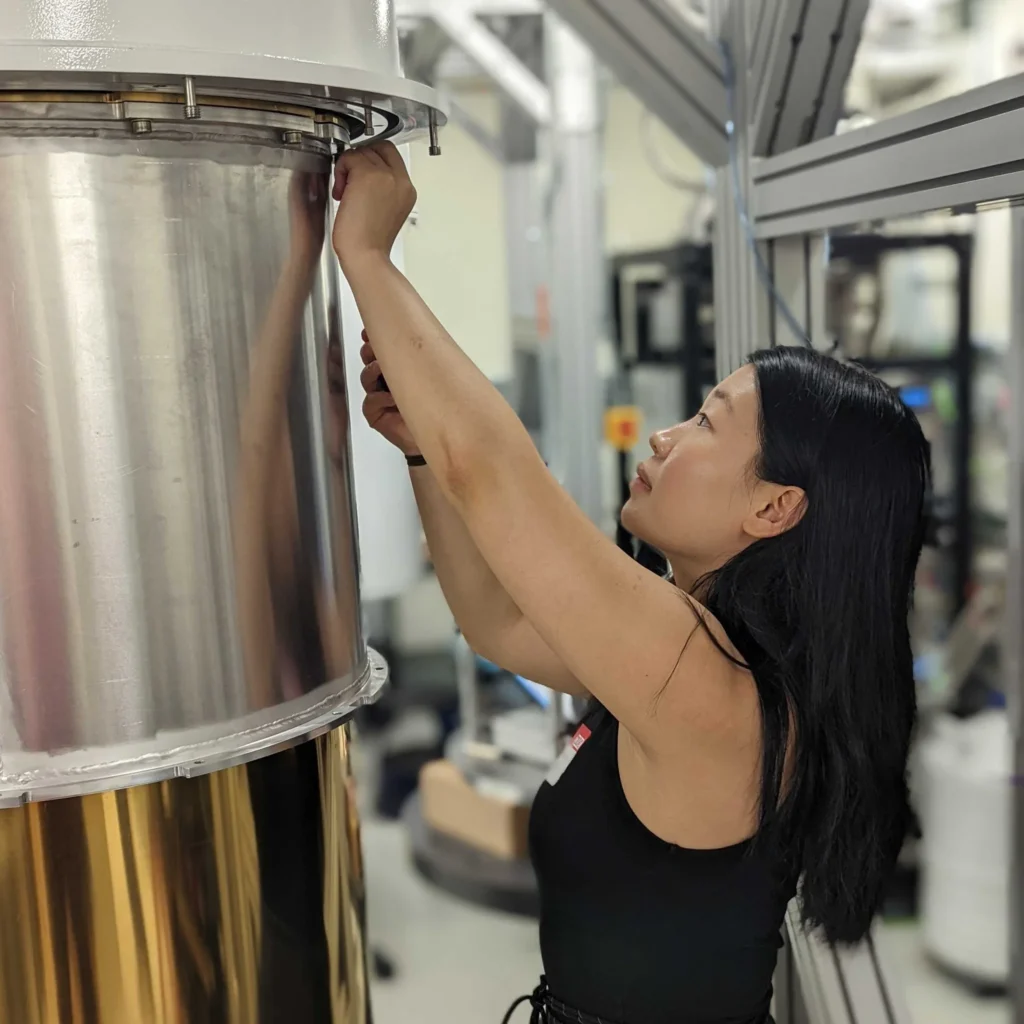
To ensure the quantum computer operates properly, it’s crucial that each of the plates is well protected and kept isolated to prevent interference from something called “black body radiation.”
Engineers achieve this by sealing the entire device in a vacuum to keep out unwanted photons, electromagnetic radiation, and magnetic fields.
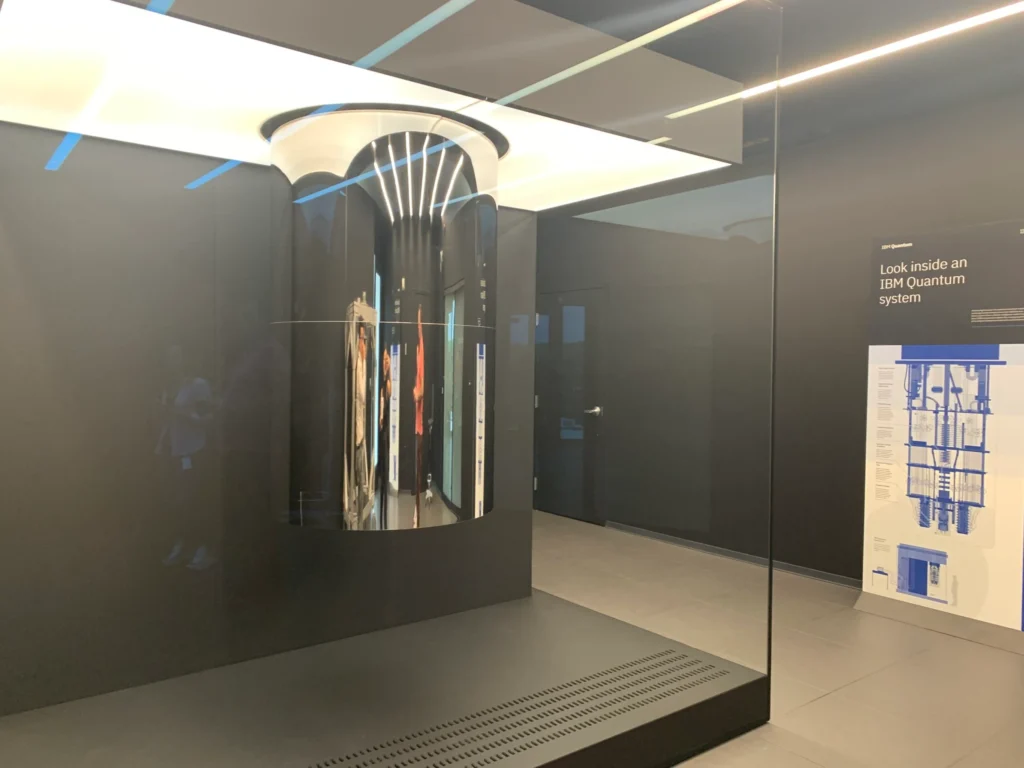
Qubits are managed using microwave signals within the frequency range of 4 to 7 gigahertz. Classical electronic systems produce microwave pulses that move through cables to deliver input signals to the chip and transport output signals back.
Along the path through the chandelier-like structure, the signal passes through components such as filters, attenuators, and amplifiers.

IBM primarily uses superconducting qubits, which are small pieces of metal positioned on wafer used to create chip. These metals are composed of superconducting materials like niobium, aluminum, & tantalum.
The key component that transforms a superconducting circuit into a qubit is a Josephson junction, created by sandwiching a very thin insulator between two superconducting materials.
Jerry Chow, who serves as the director of quantum infrastructure at IBM, explains their approach: “What we’re constructing are quantum versions of oscillators.” Oscillators convert a direct current, sourced from microwave photons in this case, into an alternating current or wave.
In contrast to typical harmonic oscillators, a nonlinear oscillator results in uneven spacing of energy levels. According to Chow, “With this, you can isolate the lowest two energy levels to represent your quantum zero and your quantum one.”
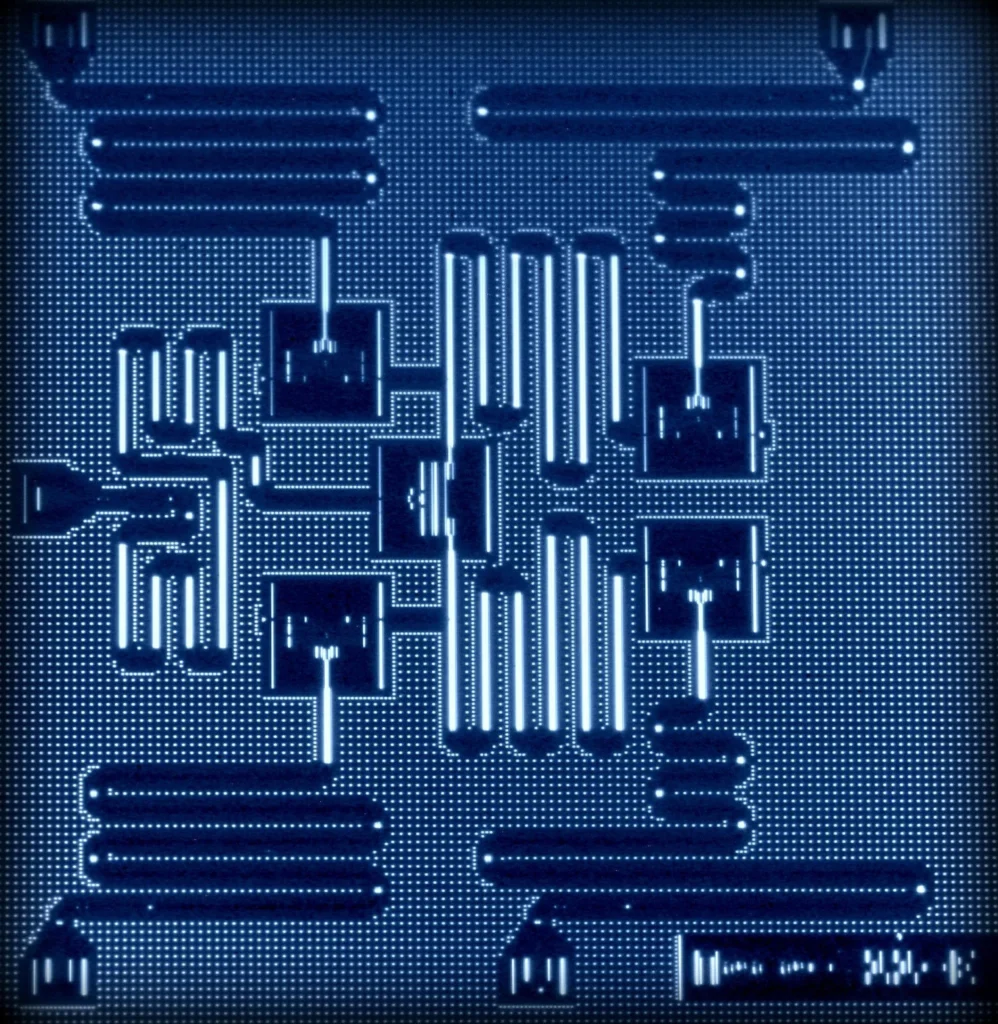
Think of a hydrogen atom in physics, which has distinct energy levels. By exposing it to the right wavelengths of light, you can make it transition between these states.
Similarly, when microwaves interact with a qubit, it functions in a similar way. Jerry Chow explains it as having “an artificial atom,” where they can manipulate a quantum of energy.
This manipulation involves applying the right amount of microwave photons in a specific manner to either excite or de-excite this quantum of energy within the nonlinear microwave oscillator.
In a classical computer, there are two states: on (one) and off (zero). In a quantum computer, the off-state is equivalent to the ground state of the artificial atom. Sending a pulse of a particular microwave photon can excite the qubit, moving it to the on-state.
If you apply the same pulse again, it returns to the off-state. Let’s say it takes 5 gigahertz for 20 nanoseconds to fully excite a qubit. If you halve either the energy or the time, you create a superposition state.
In this state, when you measure the qubit with a resonator, there’s a 50 percent chance it will be in the off-state (zero) and a 50 percent chance it will be in the on-state (one).
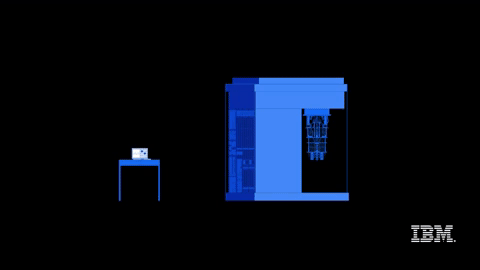
Users have the flexibility to experiment with various aspects of the circuit, such as the elements, pulse frequencies, duration, and energy, to connect qubits, exchange their states, or execute conditional operations.
This includes creating entangled states and combining individual qubit actions to achieve universal computation across the entire device.
When waves intersect, they can either enhance or break down the information being processed.
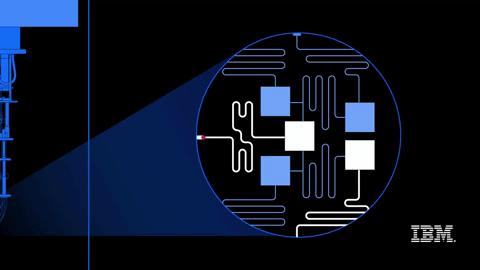
What are qubits good for?
The practical applications for quantum computers have evolved in recent years. Katie Pizzolato, Director of Strategy and Applications Research at IBM Quantum, notes that in the 2016-2018 period, quantum computing was primarily used for researching quantum-related fields like condensed matter physics and particle physics.
However, the current focus is on making experts in various fields aware of how to apply quantum computing without requiring them to become quantum experts themselves.
IBM has observed a growing interest in quantum computing for three main areas: chemistry and materials, machine learning, and optimisation (finding the best solution from multiple choices).
The goal isn’t to use quantum computing for every part of a problem but to focus on the most challenging aspects.
The IBM team is actively searching for real-world problems that are difficult for classical computers to solve due to their complexity or the mathematics involved.
Quantum computers excel in tasks like linear algebra, which involves operations like multiplying matrices and representing vectors in space.
This unique capability enables them to tackle problems like factoring, which is exceptionally challenging for classical computers due to the exponential increase in variables and their interactions.
Pizzolato highlights the potential of leveraging entanglement and other quantum features to solve these complex problems.
When it comes to chemistry and materials problems, qubits excel at simulating properties such as chemical bonds and the interactions of connected electrons.
Katie Pizzolato elaborates, “We’re considering what kinds of problems can be mapped to quantum circuits that cannot be effectively simulated using classical methods, and how we can make the most of this capability.”
“Much of the discussion revolves around how to harness the underlying mechanics of quantum devices. This involves mapping in higher-dimensional spaces and utilising the coordination and matrix multiplications to arrive at the desired solutions.”
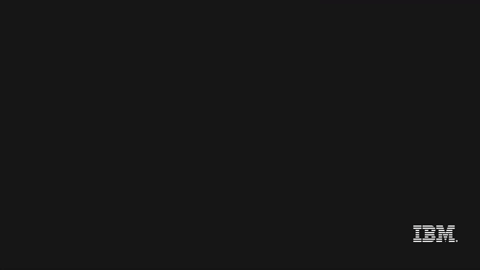
It’s important to remember that qubits can exist in states of zero, one, or a combination of both. Given that qubits are waveforms, engineers can manipulate them by rotating the zero or one to have a negative amplitude.
Qubits also have the unique property of entanglement, which has no classical counterpart. Entangled qubits can store information not only in their individual zero & one states but also in interactions between them.
Quantum circuits contain gates that can change the phase of qubits, and oscillators can entangle these qubits.
Zaira Nazario emphasises, “The art of creating a quantum algorithm lies in how you manipulate these entangled states and then interfere in a way that cancels out the incorrect amplitudes, allowing the correct ones to stand out, leading to your desired answer.”
“In a quantum algorithm, you have much more flexibility due to these entangled states and the interference they offer compared to classical algorithms, which can only toggle between zeroes and ones.”
IBM’s open-source development kit for quantum computers, Qiskit, provides information on various quantum algorithms and programs, catering to different levels of detail and expertise.
Real-world examples
If you’re finding it challenging to grasp what qubits are doing, it might help to look at some real-world examples of how IBM’s partners are applying quantum computers.
For instance, biopharmaceutical company Amgen is exploring the use of quantum computers and machine learning to forecast which patients would be most suitable for a drug trial, drawing on health records and other relevant data.
Boeing is harnessing quantum computing to analyse corrosion coefficients in aircraft. The wings of airplanes require specific material density, and engineers use different layers of materials.
However, they need assistance in determining how to arrange these layers to make the wings stronger, more cost-effective, and lighter. This challenge is essentially a combinatorial optimisation problem.
Goldman Sachs is employing quantum computing for options pricing, which involves highly complex and computationally intensive operations with intricate distributions.
This relates to calculating derivatives of variations in options, which is essentially a linear algebra operation that helps them assess risks.
In the realm of natural sciences, research groups are exploring the use of quantum computers to study photosynthesis and its underlying mechanisms.
Building in parallel
While IBM has been progressively expanding the size of its quantum processors and forging partnerships with various sectors, including industry, national government hubs, and academic institutions, the company is still in the process of determining the most effective paths for advancing both hardware and software.
IBM has previously stated its intention to create a quantum computer capable of achieving quantum advantage, which means it can consistently and accurately solve problems more effectively than classical computers, by the year 2025.
Achieving this goal not only requires the development of new components but also involves refining existing systems to enhance efficiency and address any existing challenges.
“This is a big part of the focus of the software. We’ve recognized that a lot of the tools, the error mitigation tools, the intelligent orchestration, the idea of circuit-knitting, how do we break down the problems to extend what we can do on the quantum computer, these are becoming much more prolific in how we can push the technology,” says Pizzolato.
Never miss any important news. Subscribe to our newsletter.
Related News


Tech to Reverse Climate Change & Revive Extinct Species

AI Unlocks the Brain’s Intelligence Pathways

XPENG Unveils Iron Robot with 60 Human-like Joints

Can AI Outsmart Humanity?

11 ChatGPT Prompts to Boost Your Personal Brand

Keir Starmer Hints at Possible Tax Hikes on Asset Income

Navigating the Future of AI: Insights from Eric Schmidt

AI Narrative Attacks: 3 Defense Strategies for Business Leaders

Your Health, Your Wealth

The Kashmiri Illustrator Transforming Art into Impact
